How is the decision-making center of a quantum computer physically arranged?
The most interesting part of a quantum computer is perhaps the qubit. From the point of view of materials science, how does a qubit work and what allows it to exhibit such fantastic properties?
Let's remember the most basic points that are closely related to the physics of the qubit. As usual, it’s worth starting with semiconductors. Without this, you won’t be able to feel the fundamental difference.
Semiconductor materials science
Familiar semiconductor devices work quite simply. This is a group of transistors combined into a complex architecture and outputting the expected voltage (or not).
Semiconductor transistors are often quite appropriately compared to an open or closed tap for supplying ordinary water. In this case, a faucet is a valve, which is a tool that allows you to assemble the simplest controlled logical chains.
Materials Science of Semiconductor Transistor simple. A semiconductor conducts electricity only under certain physical conditions and occupies a space between dielectrics and conductors. One of the conditions for conductivity for a semiconductor is doping – an artificial increase in the number of free electrons or vacant places for them.
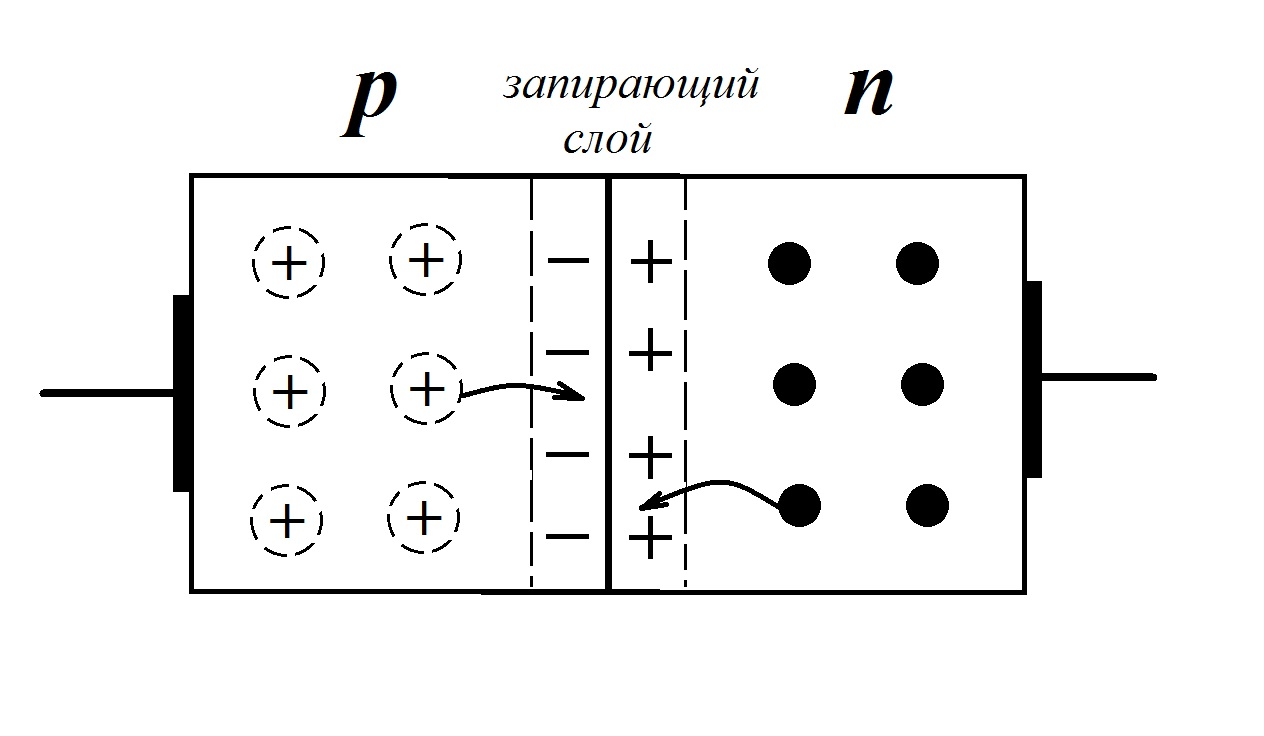
There are semiconductor materials that are “rich” in electrons, and there are materials that are “rich” in vacant places to accommodate these electrons. If you connect a material with a large number of vacancies (or holes) and a material with a large number of charge carriers (electrons) to each other, then the current will flow in one direction and not in the other. The result is a semiconductor diode.
If you combine materials according to the principle electrons – holes – electrons, then in such a triple sandwich you will get a manageable area. Applying electric current to a controlled area where there are no charge carriers will open that area and allow current to flow through the transistor. Here we could say that there are pnp or npn transistors, but this is not so important now.
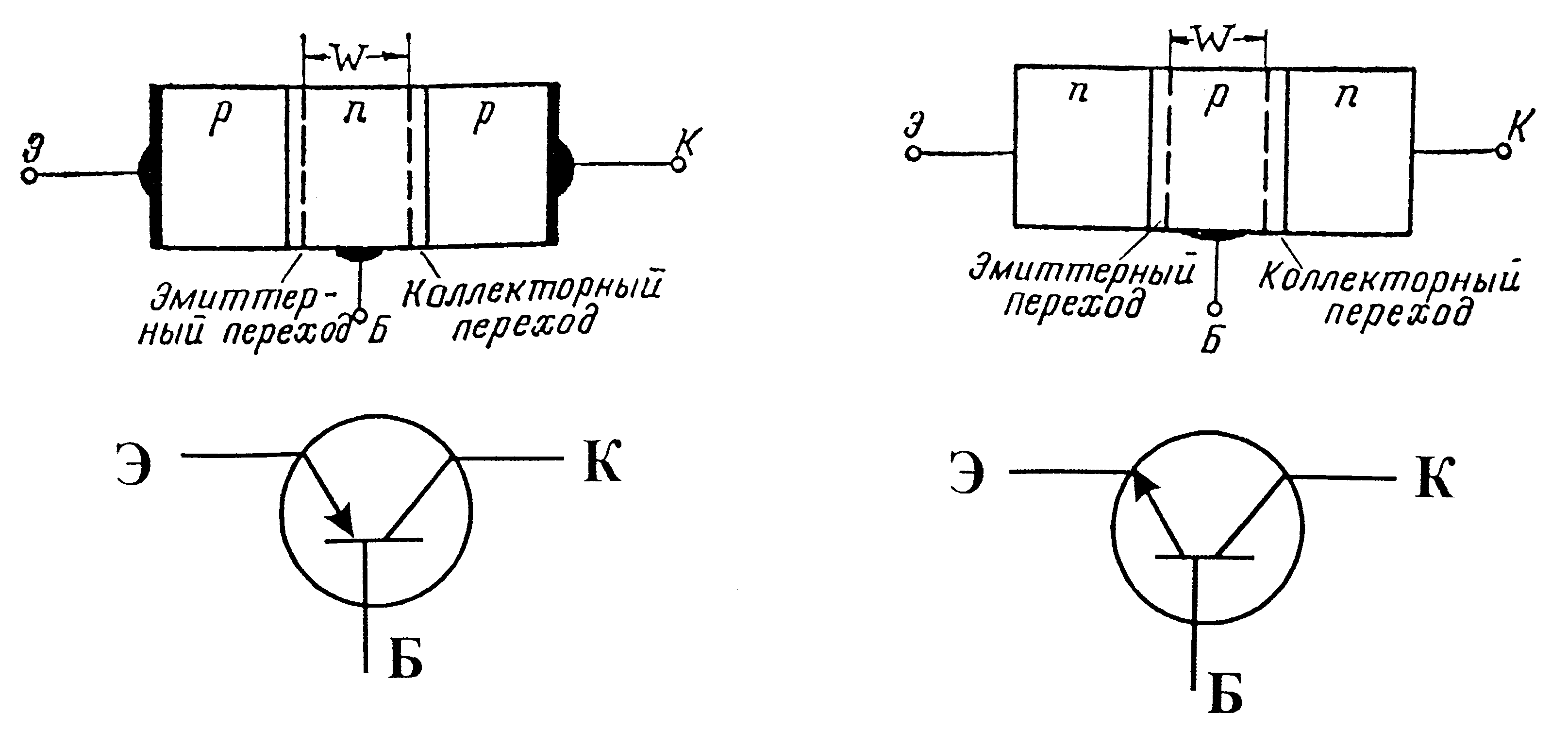
The basic principle of transistor materials science is simple. Take materials into a transistor sandwich that will provide conductivity only if electrons are added to the intermediate layer by supplying an electric current (or the diametrically opposite option for another type of semiconductor).
The main materials for transistors are germanium and silicon. In order to achieve the “abundance effect” of holes or electrons, the base material is doped. The alloying components are usually aluminum, gallium or indium. The logic is, in general, simple – add to the atoms of the main element an “impurity cuckoo”, which will penetrate into the crystal lattice of the base and introduce new free electrons into the main component (or will take over those that are in a relatively free state, having a large number of vacant places with a favorable energy transition).
We all know the result. The result is a semiconductor transistor, on the basis of which a logic cell can be assembled. Everything is simple for one reason – by and large, we are making a regular controlled water tap with yes/no positions and this falls on the usual 1 and 0. 1 and 0, if you remember, this is the bit. Strictly fixed values.
Quantum materials science
Quantum computers are known for the fact that the technology does not involve working with specific values. There are no 1s and 0s. Instead of a bit. the main element becomes cu-bit. The qubit is interesting because it can take the entire range of values from 0 to 1, and with some probability. Let's say, at a particular moment in time, a qubit can take the value “1” with a probability of 30%. As a result, the computer algorithm works with probabilistic indicators.
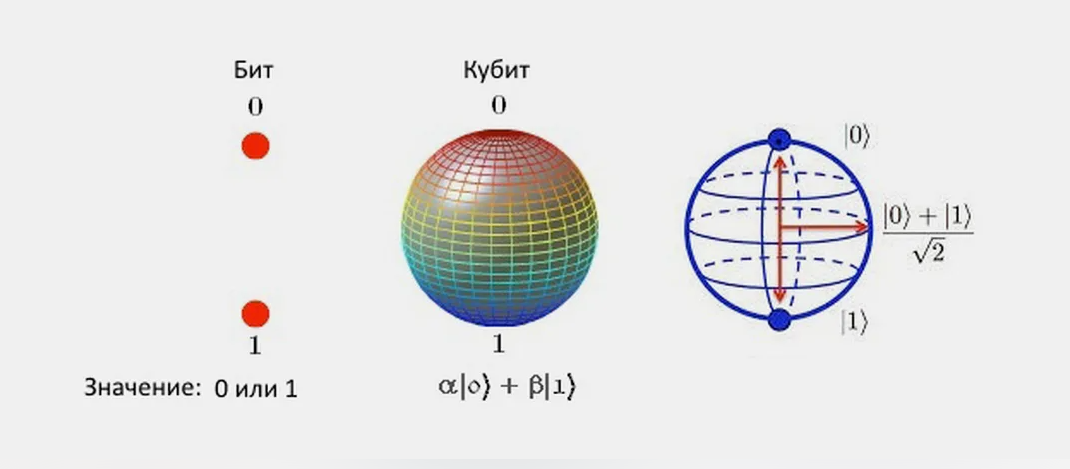
To understand you need have a good understanding of the basics of quantum physics, but I think it will be enough to understand that the qubit is always in all possible states at once. It is both 0 and 1, with all the attendant probabilities of accepting 1 and 0.
So quantum superposition manifests itself – a specific property that allows a quantum particle to take on all possible states at once. The specific value is fixed due to the observer effect – you need to measure the desired property and then the superposition will collapse, and the qubit will take one of the expected specific values.
Probabilistic calculus requires a special logic for constructing an algorithm that works completely differently from the case we are used to. As a rule, quantum algorithms are focused on working with one well-described problem. For example, forecasting changes in the temperature of some natural area. The fact of measuring a qubit will lead to fixation of the value and completion of the algorithm.
If we compared a semiconductor transistor with a controlled tap, then a qubit is something like a closed box in which anything can lie and its contents are determined by probability. You open the box with a measurement and find out the result. If you mathematically describe the probability of finding a particular result in a box, then this can be used as a way to solve some problem.
And this is where the fun begins. A how and from what to make this magic box from the point of view of materials science?
If a semiconductor computer worked like a mechanical device, then quantum qubits certainly won’t work that way. Instead of transistors, mechanical valves were initially even used, since there were only two values and they were strictly defined.
But here it is impossible to imagine a quantum computer in a mechanical equivalent. Try making a faucet that is both open and closed at the same time. Almost Schrödinger's cat. Box with a surprise.
It is necessary to operate here quantum particles, and even in a state of superposition. Just what is this from the standpoint of materials science?
In fact, it doesn’t matter what a quantum qubit is made from. The main thing is that it is something in a controlled state of superposition. The state of superposition is achieved in particles when low temperatures are created somewhere near the values of superconductivity, when all thermodynamic activity freezes. The effect of superconductivity sometimes manifests itself there.
Technically, qubits can represent a variety of different objects. Cold atoms, photons, defects in the crystal lattice. But most Superconducting qubits based on Josephson contacts are considered a popular and convenient type of qubit today.
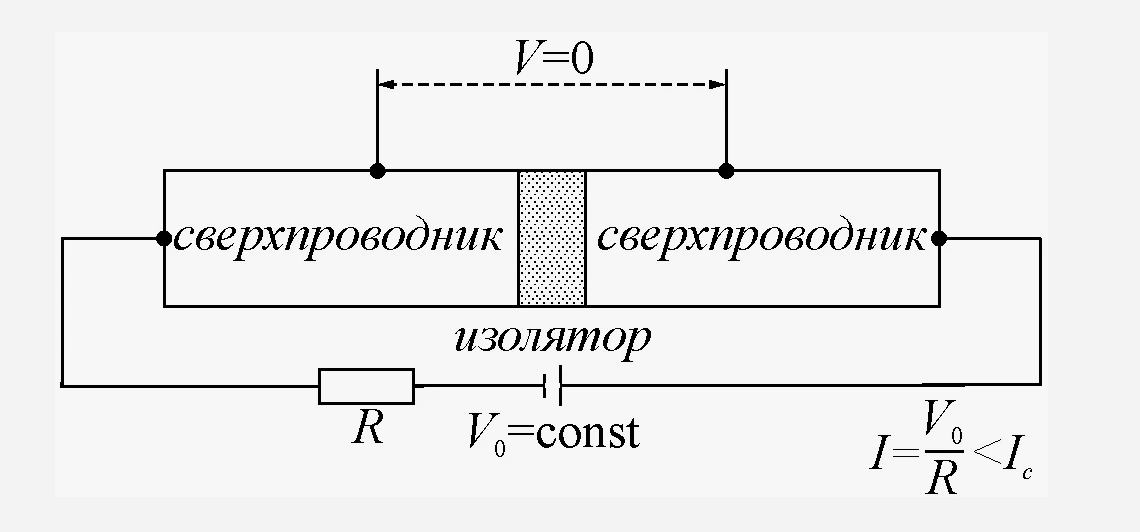
A Josephson junction is a type of connection between two superconductors through a thin layer of dielectric. You can choose different materials. Superconducting contacts can be made from aluminum, and the interlayer can be made from bismuth-tellurium-selenium or aluminum oxide.
Cooper pairs of electrons can tunnel through the Josephson junction, responsible for current transfer in a superconductor. The Cooper pair, to put it very exaggeratedly, is the electron itself and the place to place this electron in a superconductor. Through pair transfer, quantum tunneling occurs in a Josephson junction.
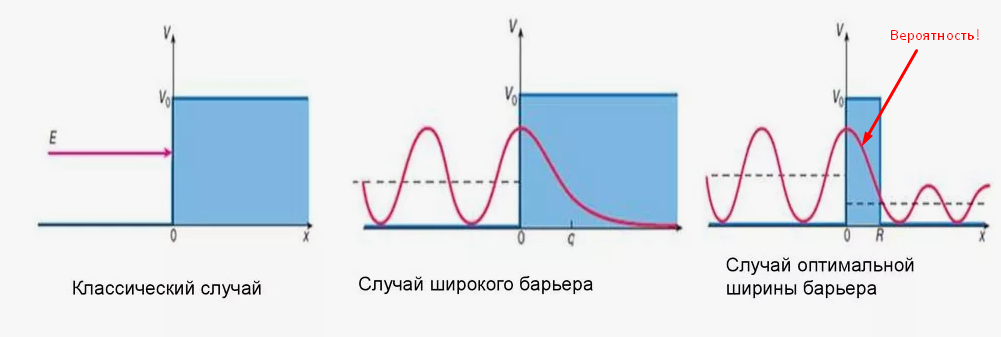
Quantum tunneling, as we remember, is an effect in which a particle can pass through a barrier when its energy is less than what is needed to jump over a high barrier. The process is probabilistic and when measuring, you can fix the position of the particle relative to the barrier. We remember that quantum tunneling is interesting because there is always a non-zero probability of being inside the barrier or appearing behind it. Remains measure the position of the Cooper pair. This is how a qubit is obtained.
We can assume that the pair is behind the potential barrier at the time of measurement with a probability of 20%. Of course, figuratively. We verify this fact by measuring and fixing the qubit.
Josephson qubits behave like individual atoms: they can be in the ground state and in several excited states, occupy several positions at once according to superposition, exchange energy through emission and absorption of photons, and even simulate laser generation modes.
Note that this is not the only approach to creating a qubit. But perhaps it is the simplest.
The fundamental difference in this whole scheme relative to the semiconductor is the complex conditions for maintaining superposition. It is this circumstance that requires maintaining a constant low temperature of the system.
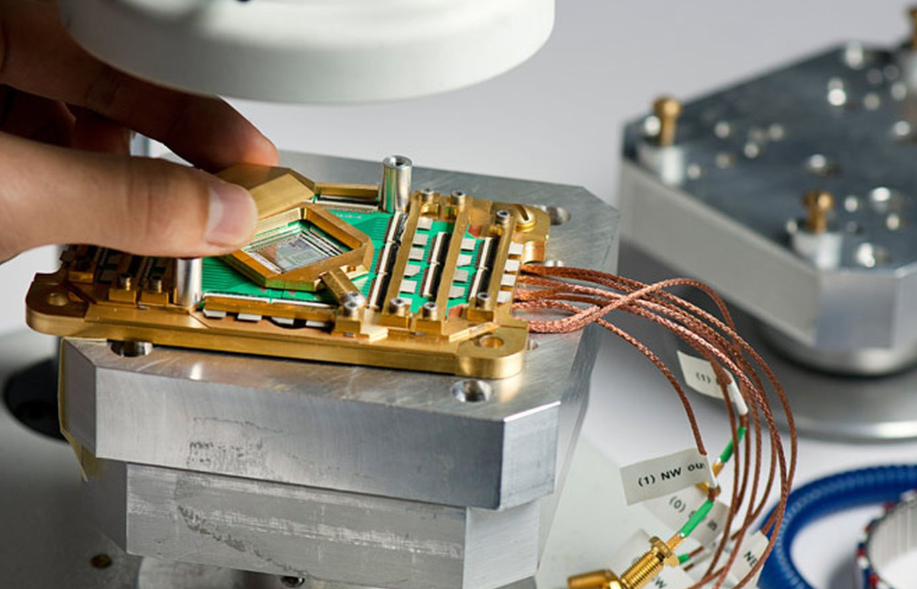
Technically a qubit (or quantum processor) consists of several thin metal plates glued together with a thin layer of dielectric. It's easy to do. It is difficult to create conditions corresponding to the state of superposition, initiate this state of the particle and maintain it for a long time. Therefore, a quantum processor is a small metal block, and together with the cooling system, the entire structure occupies a couple of rooms.
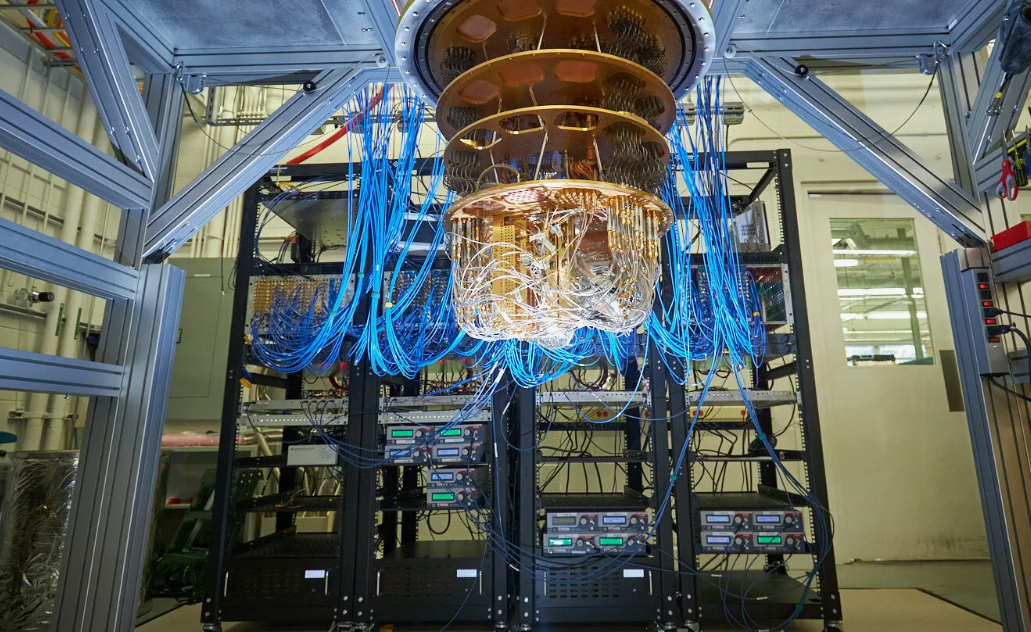
What is needed is a cooling system and a device that can, using an external electromagnetic field resonant to the transition frequency, create a superposition state between two adjacent energy levels in the qubit in question. These are complex and technically complex physical states of objects.
If you liked my article, then I invite you to also study my other notes on this topic (heading hashtag #quantumcomputer).